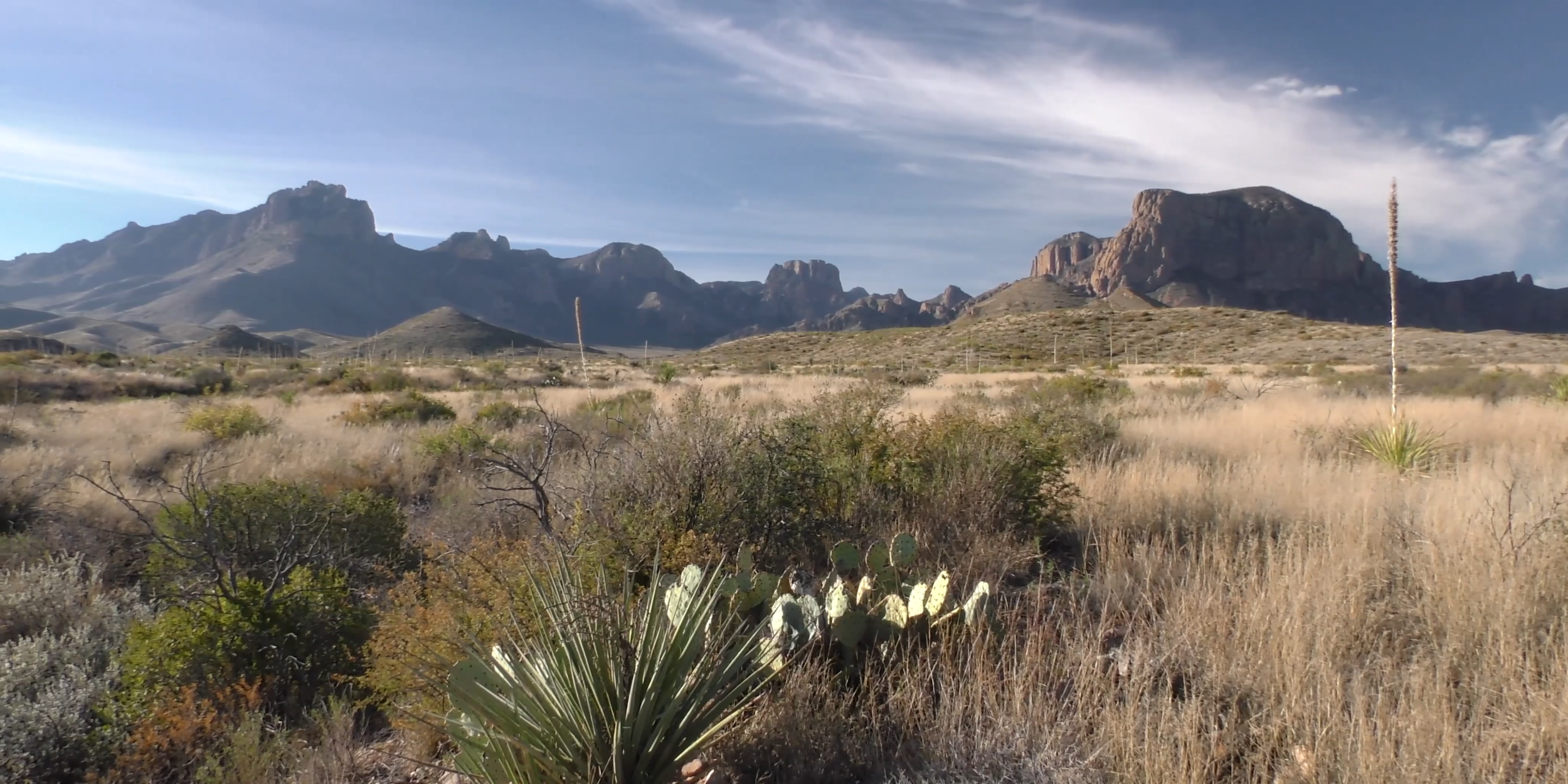
Development by Design in West Texas:
Mitigating Energy Sprawl Through Cooperative Landscape Planning
Kei Sochi, Jon Paul Pierre, Louis Harveson, Patricia Moody Harveson, David V. Iannelli,
John Karges, Billy Tarrant, Melinda Taylor, Michael H. Young and Joseph Kiesecker
May 2021
Detailed Methods: Future Energy Projections
Research conducted to understand and project energy development from oil and gas (O&G) exploration and production in the RBB study area included the broader west Texas region and the greater Permian Basin (PB) of Texas and New Mexico, which is the largest oil and gas production region in the United States [79]. We also conducted research into solar and wind electricity production in the RBB area that included the ERCOT regions of Texas but with a finer focus on Far West Texas and the potential for development in the 18-county area of the RBB project. Historically, O&G development in West Texas has experienced boom and bust cycles. Today, however, at the same time O&G activity is ongoing, new renewable energy infrastructure is being installed, sometimes colocated. Solar and wind energy infrastructure across West Texas is being built to offset the retirement of coal-fired power plants, the increased electricity demand from population centers along the I-35 corridor and the increased demand from oil field operations.
If we assume that energy development in this region continues into the future, then land alteration will also continue, regardless of the energy type. Forecasts of these alterations can be used to determine potential impacts to habitat and to aid in conservation planning, reducing long-term effects [80, 81]. Different types of energy development alter landscapes in different ways, but all of them lead to increased landscape fragmentation, which is generally defined as the loss and the breaking apart of habitat at the landscape scale [82]. Given the wide variety of landscapes and land uses in West Texas, potential impacts from land alteration need to be considered individually [83] and as a whole.
In this section, we describe the steps taken to estimate future energy development across study area. To the extent possible, we tailored a similar approach for each energy system by following a few key steps. These steps are:
Map existing infrastructure and evaluate landscape alteration patterns
Exclude anthropogenic areas restricted from development (i.e., airports, roads, cities, already developed areas)
Evaluate resource potential (i.e., reservoir quality, solar insolation, wind class)
Project amount of increased production (i.e., BOE and MW) under low, medium, and high scenarios.
Here we present detail steps for each energy system and describe differences in approaches for different energy systems, when appropriate.
1. Map existing infrastructure and evaluate landscape alteration patterns
We used the dataset published by Pierre et al (2020) [84] to map existing oil and gas infrastructure.
For solar energy, we combined and mapped data for utility-scale solar facilities from the Solar Energy Industry Association and the United State Energy Information Administration (EIA Form 860 & 860M, SEIA 2019, personal communication).
In the case of wind energy installations, we used the US Wind Turbine Database [86] (https://eerscmap.usgs.gov/uswtdb/), which is operated by the U.S. Geological Survey, the Lawrence Berkeley National Laboratory and the America Wind Energy Association. The database provides specific information on each facility, including turbine height and nameplate capacity. We assumed that all land between the turbines were part of the facility, as done by Kiesecker et al. (2019) [87], but we recognize that these interspaces often remain in use for various activities, like grazing and irrigated agriculture. We varied from the methods of Kiesecker et al. (2019) [87] and used a concave hull to circumscribe the perimeter boundaries of existing wind farms (string or multiple strings of turbines), and then calculated the median area of these polygons to represent the amount of land that would be impacted from future wind farm development.
2. Exclude anthropogenic areas restricted from development (i.e., airports, roads, cities, already developed areas)
For all energy types, we followed the same process described by Pierre et al. (2020)[84], who compiled information from 18 datasets (Table S5), including those showing cemeteries, waterbodies (including all stream orders, rivers, lakes, etc.), protected areas, roads, national and state parks, etc. For each energy type, existing facilities were excluded as possible locations for future infrastructure, though we did allow for future colocation of different energy types.
3. Evaluate resource potential (i.e., reservoir quality, solar insolation, wind class)
In the case of O&G development, we projected future energy development by determining the trends of individual well pad development [84] and well-by-well energy production, attributed to each well pad, over the last 10 years, aggregated at the 1 km2 scale. If the number of well pads increased with time and if energy production from existing or new wells increased with time, these would indicate that operators were accessing productive rock and that future well pads would be needed to support future drilling (given favorable economics). In these cases, we extrapolated the number of expected new pads forward in time by 33 years (from 2017 to 2050), and assigned these pads to specific 1 km2 areas, except where excluded by existing infrastructure or other features (see [84]).
In the case of solar development, we tailored the guidelines set forth by Wu et al. (2015 [88], see Table S2) to data available for Texas. This included mapping of available (not excluded) contiguous regions at least 1 km2 or greater, with a global horizontal irradiance (GHI) of at least 5 kWh/m2/day or more, and with a slope of 3% or less. The GHI data were obtained from the National Renewable Energy Laboratory (NREL, 2020, which cited [89]).
In the case of wind development, we also tailored the guidance set forth by Wu et al. (2015)[88]. This included identifying contiguous regions at least 8 km2 in area with a slope of 20% or less. However, due to wind data limitations, we followed NREL recommendations and used wind speeds 6.5 m/s or greater [90]. Using these criteria for solar and wind, we could identify lands we considered “suitable” for future utility-scale development.
4. Project amount of increased production (i.e., BOE and MW) under low, medium and high scenarios
To cover the range of uncertainty that accompanies any forecast, we identified three landscape impact scenarios for O&G, solar and wind energy. Impact in this report refers to the construction of well pads, pipeline right-of-ways (ROWs), photovoltaic solar installations, etc., all of which accommodate energy production. The approach used for the different energy systems was made as consistent as possible between energy sources. In each case, we selected a medium impact scenario, which we assumed was the business as usual (BAU) case, essentially following along existing trends. We then bracketed the BAU case with low and high landscape impact scenarios. Specific differences between the three energy systems are described:
For O&G development, we assumed that three wells per pad (on average) was a reasonable expectation for the future ([91, 92] and multiple discussions with various operators), and thus we considered this our medium landscape impact (BAU) scenario. Additional justification for three wells per pad can be found in Pierre et al. (2020) [84], where we note that historical well densities are closer to 1.15 wells per pad across the Permian Basin [12]. Thus, if future development follows the same, low well density practices, then more pads will be needed to accommodate the new wells, hence leading to a high landscape impact scenario. To develop a low landscape impact scenario where fewer well pads will be needed, we assumed either reduced well-drilling activity given low O&G prices, or an increased number of wells per pad as a result of technological advances or other economic incentives, or both. Operationally, this would triple the number of wells per pad (compared to the BAU case) to nine wells per pad (hence, 1/3 the number of well pads would be needed).
To match electricity demand anticipated by ERCOT for the next 30 years, with future generation capacity, RBB contracted with IdeaSmiths, LLC, an Austin-based company (https://www.ideasmiths.net/) that conducted modeling to assess future capacity needs. IdeaSmiths took into account factors that would influence renewable energy needs (e.g., retirement of coal plants, increases in population, economics of natural gas) and possible locations for the infrastructure based on the above-mentioned wind and solar resource maps available from NREL. The outcome of their modeling provided future estimates of both solar and wind generation, in megawatts (MW), for each county in Texas.
For solar and wind energy production, future development was estimated together in a single model to take advantage of differences in peak performance between day (solar) and evening (wind) time periods. The model included facilities across the entire state of Texas, which reduces uncertainty of modeling the west Texas region in isolation (electrons move into and out of west Texas but not across state lines). The Capacity Expansion Model, known as “Switch,”[26]was used for this project, using the following input values that separated medium (BAU), low and high impact scenarios.
Electric Vehicle Demand = ERCOT LTSA Forecast (ERCOT 2018)
Distributed Solar Capacity = ERCOT LTSA Forecast (ERCOT 2018)
Wind/Solar/Battery Capital Cost = NREL ATB 2019, Low (NREL 2019)
Natural Gas Combined Cycle Capital Cost = NREL ATB 2019 (NREL 2019)
Coal/Gas Prices = EIA AEO 2020 Prices (USIEA 2020)
Transmission Capital Cost = CREZ Project Data (Deetjen et al. 2018)
Electric Vehicle Demand = BAU X 0.5
Distributed Solar Capacity = BAU X 1.5
Wind/Solar/Battery Capital Cost = NREL ATB 2019, Mid (NREL 2019)
Natural Gas Combined Cycle Capital Cost = BAU
Coal/Gas Prices = BAU X 0.75
Transmission Capital Cost = BAU X 1.25
Electric Vehicle Demand = BAU X 1.5
Distributed Solar Capacity = BAU X 0.5
Wind/Solar/Battery Capital Cost = BAU X 0.75
Natural Gas Combined Cycle Capital Cost = BAU X 1.25
Coal/Gas Prices = BAU X 1.25
Transmission Capital Cost = BAU X 0.75